ONGOING PROJECTS
- Development of Remote Radiation Detection Imaging System Mounted on a Drone
- High Resolution SPECT using Variable Pinhole Collimator
- Positioning Algorithm for CZT Virtual Frisch-grid Detector
- Multi-purpose Super-resolution Gamma Detector
- Multi-pinhole SPECT
- Low Profile Light Guide using Diffusion Film
- Advanced Reconstruction for Radiation imaging (ARRA)
COMPLETED PROJECTS
- Reconstruction of Dose Distribution in In-beam PET for Carbon Theraphy
- Image Registration for Breast Cancer Study
- High Energy Collimator Design for I-131
- Plasma-Display-Panel based X-ray Detector (PXD)
- CCD based Gamma Camera
- Abdomen Registration for PET/CT and MR images
- Performance Optimization by Patient Dose Analysis and Image Quality Assessment in CT Fluoroscopy
- Cone-beam based system matrix for a pixelated SPECT detector
- Development of Time-of-Flight Method for Improvement of Signal-to-Noise Ratio
Limited Angle Gamma Imaging for Small Objects Using a Multi-pinhole Collimation System
Introduction
Gamma imaging systems used in the single photon emission computed tomography (SPECT) have now been developed to have a smaller footprint, faster scan time, and organ-specific applicability. Detectors of small-footprint systems are required to have small dimensions but high resolution to implement a small field of view (FOV). Detector sensitivity should be high for it to be used in a fast scan time system. The organ-specific system needs to be focused on a specific organ, such as the thyroid or heart. The movement of the gantry needs to be optimized with respect to the target organ for obtaining a focused image so that the gantry geometry to become simplified.
Because of the high magnification characteristics of a pinhole collimator, it is possible to achieve high intrinsic spatial resolution. Due to the excellent spatial resolution characteristics of a single-pinhole collimator, the collimator is applied in preclinical SPECT for small object imaging. However, the sensitivity of a single-pinhole (SP) collimator is very poor. As a result, the scanning time is long, becoming a significant disadvantage in small object imaging and clinical studies. To overcome these drawbacks of a SP apparatus, a multi-pinhole (MP) collimator has been used and studied by many groups. A multi-pinhole collimator has several pinhole apertures to increase the system sensitivity so that the acquisition time and patient dose are reduced. MP collimators have a reduced magnification factor, which is caused by the separation of the detection area and induces degradation of the spatial resolution when compared with SP collimators. Incomplete sinogram data are acquired to simplify the gantry geometry. We applied a limited angle reconstruction technique to compensate for the lack of angle coverage.
The goal of this study was to design and evaluate an MP collimator for a small footprint, fast scan time, and applicability to organ-specific systems, which would require a small FOV, a high spatial resolution, a high sensitivity, and simple gantry characteristics. The MP collimator was used to obtain a better spatial resolution and higher sensitivity than SP collimator. Limited angle reconstruction with different angle coverage and angle intervals (less than 180°) was explored to overcome the limitations of the gantry movement.
The organ-specific SPECT systems which has been commercialized or currently under development have mostly focused on heart imaging. In addition, the heart is slightly off-centered from the body symmetry. If we scan the heart with a small-FOV detector from the closest distance and within limited angle coverage, we can effectively evaluate the feasibility of the proposed multi-pinhole design. Therefore, we focused on the heart as a target organ for this study, although our approach is not limited only to heart imaging.
Materials and Methods

Fig. 1. Schematic illustration of the proposed pinhole collimator. (A) A single-pinhole collimator, (B) a multi-pinhole collimator, and (C) a top view of the area (dark gray) that is covered by the multi-pinhole collimator.
Our group has been developing a high resolution, small field of view (SFOV) gamma detector, which has an FOV of 200 mm × 140 mm. The dimensions of the detector are 270 mm × 200 mm, and its intrinsic resolution is 3 mm. A conceptual sketch of the collimator is given in Fig. 1. In the SP collimator, a pinhole has a diameter of 2 mm with an acceptance angle of 60°, and the collimator thickness is 4 mm. Each pinhole has a cylindrical shape. The thickness of the collimator is adjusted to obtain the designed acceptance angle in the SP collimator. The MP collimator consists of eight pinholes (each pinhole has a diameter of 2 mm), and the collimator thickness is 3 mm. The projection area that is covered by a pinhole is 50 mm × 50 mm, which falls on exactly one-eighth of the entire FOV. Two different pinhole configurations, inner and outer pinholes, were developed to avoid the multiplexing effects between adjacent FOVs. In Fig. 1 (C), pinholes numbered (1), (4), (5), and (8) are the outer pinholes that have an acceptance angle of 48.1°, whereas pinholes numbered (2), (3), (6), and (7) are inner pinholes that have the acceptance angle of 52.2°. All the pinholes were tilted towards the center of the FOV in order to maximize the collimator efficiency.
Simulation models for SP and MP collimators were constructed by using a geant4 application for the tomographic emission (GATE) tool. We used two types of physical phantoms: the three spherical phantoms and the heart phantom. First, the three spherical phantoms which passed through the center of the FOV (transverse slice) were generated in the GATE simulation with the designed SP and MP collimators. Spherical phantoms were close to the detector surface so that each the diameter of each phantom was 3 mm and the intervals between them were 30 mm. The activity of each source was 0.5 mCi and the background activity was 0.1 mCi. The acquisition time was 60 seconds per angle. Second, a heart phantom was generated by X-CAT. The pixel size of the generated phantom was 0.625 cm, and the slice size of the generated phantom was 0.625 cm, with 41 arrays and 41 slices. The activity of heart phantom was 0.5 mci and the acquisition time was 10 seconds per angle. Moreover, the three spherical phantoms and the heart phantoms were used for the limited angle reconstruction. Simulation studies provide coverage data for the angles of 60°, 90°, 120°, 150°, and 180°, with different angle intervals (3°, 6°, 9°, and 12°).
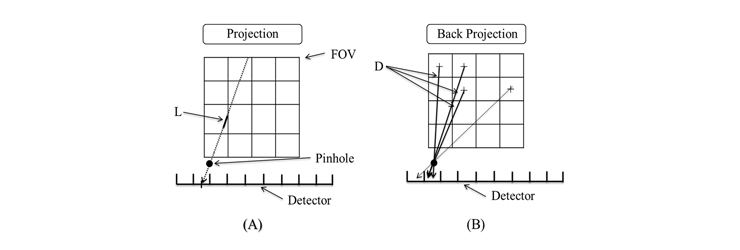
Fig. 2. Unmatched system model: (A) projector and (B) back-projector.
Results

Fig. 3. Projections and reconstructed images of single-pinhole and multi-pinhole collimators(left), Profiles of reconstructed images(right).
Fig. 3 shows a projection of three spherical phantoms acquired by the simulation and the reconstruction images through the angle coverage of 180°, in intervals of 3° (61 projections). There were 3,728 counts per projection of a SP collimator and 22,367 counts per projection of a MP collimator. The FWHMs of a center source around the SP and MP collimators were 6.25 and 7.18 mm, respectively. The sensitivity of the MP collimator was more than six fold greater than that of the SP collimator, while the resolution was preserved in the acceptable range of decreases.
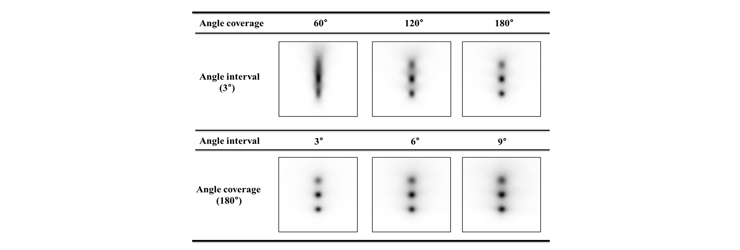
Fig. 4. Results of the angle coverage and angle interval studies for 3 spherical phantoms. In the top panel, the images were reconstructed with the angle interval of 3°, and 8th iteration shows the results obtained for different values of angle coverage. In the bottom panel, the images were reconstructed with different angle intervals and an angle coverage of 180°, and 8th iteration shows the influence of the angle interval.
Fig. 4 shows the reconstruction images that were obtained using 3°, 6°, 9°, and 12° angle intervals and 180° angle coverage of the reconstruction data set, and it also shows the reconstruction images obtained using 60°, 90°, 120°, 150°, and 180° angle coverage. The profiles of these images are compared in Fig. 5.
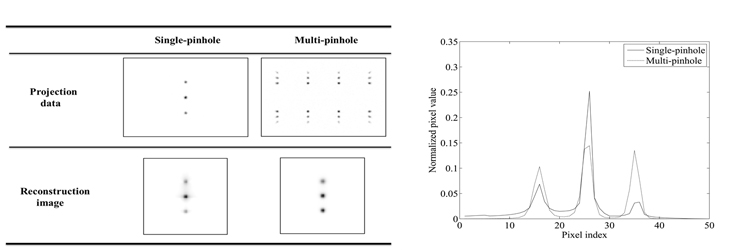
Fig. 5. Profiles obtained for the angle coverage and angle interval results in Fig. 9. (A) Angle coverage profile results, and (B) angle interval profile results.
The angle of coverage of 120° and the angle interval of 6° (21 projections) appear to constitute optimal parameters for the limited angle reconstruction with respect to projection numbers and image quality. The optimal parameters of the proposed MP collimator were used one-third of projections. These results also indicate that a change in the angle coverage affects the degradation of image quality more significantly than a change in the angle interval. This suggests that the angle coverage is more critical than the angle interval in determining the quality of the reconstructed image. The target system of the proposed MP collimator required minimal gantry movement, the angle coverage of 120° and the angle interval of 6° were chosen as the cost-effective and efficient parameters of the proposed MP collimator. Reconstruction results obtained using those parameters are shown in Fig. 6.

Fig. 6. Reconstruction image of (A) 3 spherical phantoms and (B) X-cat phantom, obtained for the angle coverage of 120° and angle interval of 6°.
The three reconstructed spherical phantoms that are shown have 12.18 mm FWHM, that was quite increase but this resolution was still sufficient to distinguish between these spherical phantoms. The heart phantom appeared to have a satisfactory image quality. The cost-effective parameters of the proposed MP collimator were used one-third of projections.
Conclusion
The purpose of this study was to design a gamma imaging collimator for small foot print, fast scan time, organ-specific applications. For the small foot print and fast scan time system, we investigated a MP gamma collimator for the SFOV gamma camera and generated a 3D imaging method based on the geometry of the collimator. For realizing an organ-specific system, we studied a limited angle reconstruction. We performed systematic simulations using the GATE simulating tool in order to demonstrate the feasibility of our collimator system, and these studies showed promising results. Results of our studies show that the MP collimator system has six times higher resolution as compared to the SP collimator system that is 0.9 times smaller. The quality of the reconstructed images is improved as a function of the number of iterations and projections, and there was an optimal set of parameters: the angle interval of 6°, angle coverage of 120°, and 8 iterations. With these parameters, it was possible to distinguish the 3.0 cm interval spherical phantoms with 12.18 mm FWHM. Our designed MP collimator was found suitable for realizing a small foot print and fast scan time system because it achieved reduced scan time with an adaptable system resolution. Moreover, the use of the limited angle reconstruction technique makes the system more efficient and robust for organ-specific applications such as thyroid, heart, breast, etc.
Participating Researchers